QCD Studies
The Large Hadron Collider (LHC) is by now fully operational and producing fascinating data on proton-‐ proton (pp), proton-‐nucleus (pA) and heavy ion (HI) collisions at center of mass collision energies an order of magnitude higher compared with the previous largest nuclear collider RHIC. This energy range has been terra incognita over the last decades, and now the experiments at LHC are exploring this new area, providing data to be explained and formulating numerous challenges for the contemporary theory of strong interactions, quantum chromodynamics (QCD).
.
Hard QCD collisions at ATLAS
Here is a selected list of the hard QCD reactions measured at ATLAS, and of the observed effects, which we plan to work on within the ANILLO project. Proposed new measurements are presented as well
P-P Collisions
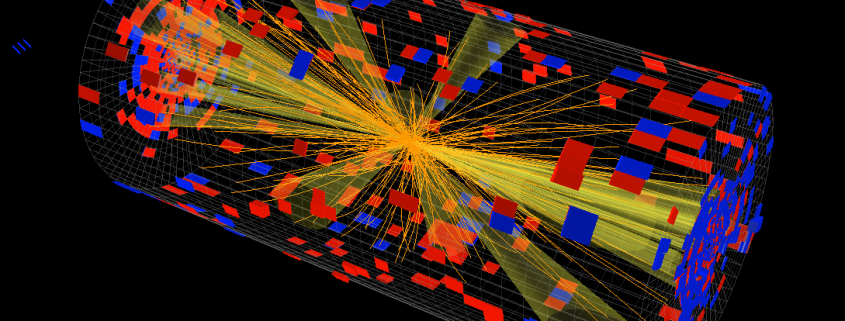
PP Collision at the LHC
- The ATLAS experiment has performed an intensive study of heavy quarkonia (J/ψ,ψ(2S), χc, Υ) in pp collisions at = 7TeV and high transverse momenta [1, 2, 3, 4, 5]. Some of our experimental group members (Will Brooks, Miguel Arratia) are particularly working on charmonia. For the members of the theory group heavy quarkonia has also been one of the favorite topics. While production of χ states [6] and photoproduction of charmonia [7] were well explained, charmonium production at high p is still under debate. The popular color-‐singlet model badly failed to explain high-‐pT data of the CDF experiment at the Tevatron, and no proper alternative has been proposed, except the model in [8], which, however, also faces problems explaining data. We plan to revisit the color singlet model and perform calculations with more developed theoretical tools, within the color-‐dipole phenomenology. We also plan to calculate the contribution of multi-‐ gluon emission, which we expect to dominate the high pT range.
- Measurements of pT -‐broadening of charmonia produced in very high multiplicity pp collisions is proposed in [15], relying on the analogy with pA collisions. The latter can also be measured in the ATLAS experiment.
- ATLAS has measured inclusive production of Drell-‐Yan pairs [9] and gauge boson [10] in pp collisions. Measurements of the same processes in the diffractive channel was proposed and predicted in [11 12]. A prominent feature is expected strong breakdown of widely believed diffractive factorization. Measurements of these diffractive reactions at ATLAS seemto be feasible. Diffractive production of Higgs via the intrinsic heavy flavors also was predicted in [13], and more recently in [14] by the higgsstrahlung mechanism, calculated specifically for the ATLAS kinematics.
- An important ingredient of the proposed new measurements is the efforts of our experimental group in manufacturing the part of the ATLAS spectrometer called Small Wheel. It is is designed to detected muons, which are the decay products of all particles listed above, char-‐ monia and bottomia, Drell-‐Yan pairs, Gauge bosons W and Z. The Small Wheel detector is an essential part of the present proposal (see below).
P-A Collisions
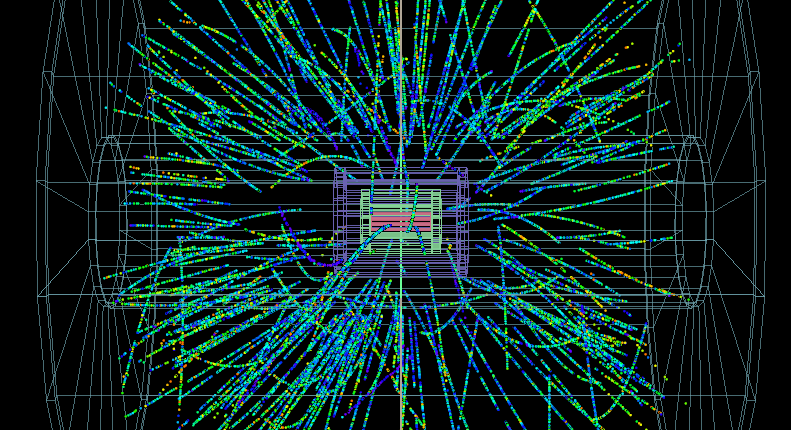
PA Collision at the LHC
- Measurements by ATLAS of multi-‐particle high-‐pT correlations in proton-‐lead collisions revealed strong effects [16, 17, 18], which have not been properly explained so far. Such correlations, of a rather large magnitude, should be expected within the model, proposed in [19, 20]. The model is based on the dipole phenomenology and includes the parton saturation effect. It contains correlation between the dipole orientation and the impact parameter of collisions. As a consequence, all gluons radiated in the sane event must correlate with each other, and such correlation is long-‐range, what indeed was observed in data. We plan to interpret the ATLAS observations employing this model.
- Data on charmonium production in proton-‐lead collisions at ATLAS are expected soon, and the nuclear effects were predicted in [6, 21]. However, what was actually calculated were the nuclear effects for χ2 production, which are expected to be similar to J/ψ. We plan to perform new calculations specifically for J/ψ, as far as the production mechanism in pp collisions will be settled (see above).
A-A Collisions
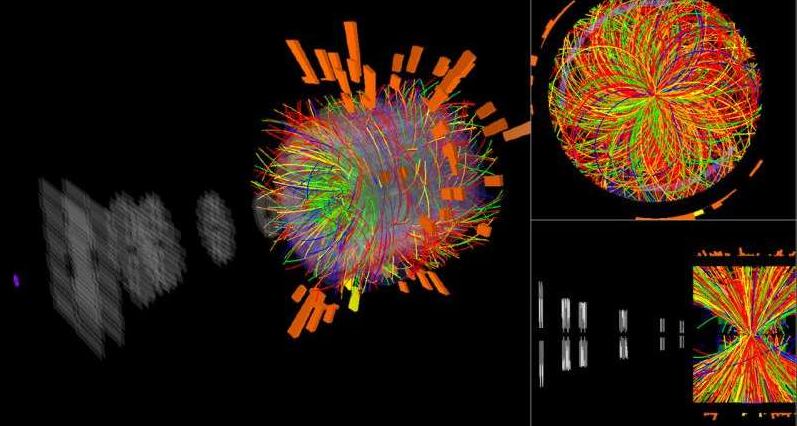
AA Collision at the LHC
- Charmonium production in heavy ion collisions is a popular probe for the properties of the created hot matter, which effectively dissolves and suppresses charmonium states [22]. A considerable progress in interpretation of final-‐state interaction (FSI) effects was done recently in [23]. We plan to perform full calculations, including initial-‐state interaction (ISI) suppression [21], for J/ψ, ψ(2S) and Υ. Azimuthal asymmetry parameter v2 will be calculated as well.
- We plan to extend our previous studies of jet quenching in HI collisions for single hadron production [24, 25] to suppression of jets. First of all, we plan to develop the method of calculation of the time-‐ dependent energy loss proposed in [26] to explain the observed correlation between the magnitude of suppression of a jet and its radius [27]. We expect such a correlation to be strong due to the vacuum dead-‐cone effect found in [26]. This effect is also expected to lead to a similar suppression of heavy flavor and light quark jets at sufficiently high pT .
Soft QCD processes at ATLAS. Saturation phenomena
- The main observable characterizing the soft interaction dynamics is the elastic scattering cross section, which is related via the unitarity relation with all soft-‐dominated inelastic collisions. With the sub-‐detector ALPHA of ATLAS the elastic pp scattering cross section was measured with an extraordinary precision at s = 7 TeV [28]. Among the numerous attempts to predict it in this energy range, the 14-‐years old calculations [29, 30] turned out to demonstrate the best agreement [31]. The basic ingredient of this model was a short range of gluonic interactions, dictated by data on diffractive dissociation and by many other sensitive observables [32]. Presence of such a semi-‐hard scale in the proton allowed us to calculate the energy dependence in a parameter-‐free way (although with an uncontrolled accuracy), and predict its rate to rise with energy. Since the ATLAS measurements extend down to very small transverse momenta, as low ast ≈ 0.005GeV2, this allows performing another stringent test of the model. We plan to perform a comparison with our predictions including Coulomb contribution. We will employ in particular the recent updates for the Coulomb phase [33].
- At a soft scale (boosted up by saturation) both the quest for new physics in pp collisions and investigations of the medium effects in HI collisions require a solid understanding of multi-‐gluon interactions. Gluon densities rise rapidly with energy, as has been discovered at HERA. Whenprobed at very high energies, or equivalently, at low Bjorken x, the proton resembles a dense cloud of gluons. The low x rise of the gluon density is driven by the linear BFKL equation [34] unless the non-‐linear effects become important. These effects slow down the growth rate of the density, which eventually saturates [35] resulting in appearance of a new scale Qs(x) known as the saturation momentum, which might be rather high. Such a dense gluonic state is frequently referred to as Color Glass Condensate(CGC) [36]. The QCD coupling αs, evaluated at the saturation scale Q2s = 5-‐10 GeV2 for the LHC energies, is small, which makes possible to apply the weak coupling methods. Nevertheless, the high gluonic density leads to a breakdown of the naive perturbation theory. This is analogous to the situation with an atom in a strong external field, where the smallness of the electromagnetic coupling is compensated by a large ion charge Z, and the higher order corrections must be re-‐summed in all orders. A non-‐linear generalization of the BFKL equation, which resums the high-‐density effects, is known as the BK-‐JIMWLK functional equations [37, 38, 36, 39]. These equations are valid when one of the colliding particles is dilute, e.g. in deep inelastic scattering (DIS), or (with a limited accuracy) in proton induced reactions. This is certainly not the case for heavy ion collisions. The behavior of such systems at high energies is still not understood well.
- One of the goals of this project is toachieve further progress in developing the theory of high-‐energy collisions beyond the BK-‐JIMWLK approximation, in order to improve the interpretation of already avail-‐ able data from LHC, in particular from ATLAS, and propose new measurements.More specifically, we plan to work on attempting at a solution of the longstanding theoretical problem in CGC/saturation approach: the large impact parameter behavior of the partial elastic amplitude, which should decrease exponentially as exp(−μb), is in conflict with perturbative QCD, including the CGC/saturation model, which predict a power dependence on b [40, 41]. A solution of this problem will allow to develop a better grounded description of soft physics at ATLAS, in particular of the elastic and diffractive cross sections.
- We plan to establish a relation between BK-‐JIMWLK functional equations and the BFKL Pomeron calculus. The advantage of the latter is a possibility to be applied to dilute-‐dilute (proton-‐proton), dilute-‐ dense (proton-‐nucleus), and dense-‐dense (heavy ions) systems of par-‐ tons. The current status of this challenge can be found in Refs. [42, 43, 44, 45, 46] A solution of this problem wil allow to improve the theoretical treatment of HI collisions, which at present is based on the BFKL Pomeron calculus. In particular, we plan to perform a proper description the ATLAS data [47] for inclusive and double inclusive particle production in HI collisions in the CGC/saturation domain.
- A novel mechanism for long-‐range azimuthal correlations, based on variations of the density was proposed in [48, 49]. We plan to investigate numerically whether this new source of correlations can explain corresponding data from ATLAS [50, 51, 52].
- ATLAS data for single isolated photon [53] and double photon [54] production canbe interpreted in terms of the light-‐cone dipole description. Corresponding theoretical studies were performed in [55, 56, 57] and in [58] for single and double photon radiation respectively. One of the main shortcomings of such an approach is rather large theoretical uncertainty, due to a limited kinematics of available low-‐ x data, used for phenomenological fits. The color-‐dipole amplitude extracted from global analysis is not sufficiently constrained [59, 60]. Moreover, higher order corrections beyond the leading-‐log approximation are expected to be important at the LHC kinematics and they should be considered for a better understanding of data. We plan to improve previous calculations beyond the current leading-‐log approximation, and to provide predictions for different observables in the upcoming new ATLAS measurements.
References
[1] G. Aad et al. [ATLAS Collaboration], Nucl. Phys. B 850, 387 (2011).
[2] G. Aad et al. [ATLAS Collaboration], JHEP 1409, 79 (2014).
[3] G. Aad et al. [ATLAS Collaboration], JHEP 1407, 154 (2014).
[4] G. Aad et al. [ATLAS Collaboration], Phys. Lett. B 705, 9 (2011).
[5] G. Aad et al. [ATLAS Collaboration], Phys. Rev. D 87, no. 5, 052004 (2013).
[6] B. Kopeliovich, A. Tarasov and J. Hufner, Nucl. Phys. A 696, 669 (2001).
[7] J. Hufner, Y. .P. Ivanov, B. Z. Kopeliovich and A. V. Tarasov, Phys. Rev. D 62, 094022 (2000)
[8] V. A. Khoze, A. D. Martin, M. G. Ryskin and W. J. Stirling, Eur. Phys. J. C 39, 163 (2005).
[9] G. Aad et al. [ATLAS Collaboration], Phys. Lett. B 725, 223 (2013).
[10] G. Aad et al. [ATLAS Collaboration],G. Aad et al. [ATLAS Collaboration], JHEP 1012, 060 (2010); Phys. Rev. D 85, 012005 (2012).
[11] R. S. Pasechnik and B. Z. Kopeliovich, Eur. Phys. J. C 71, 1827 (2011).
[12] R. Pasechnik, B. Kopeliovich and I. Potashnikova, Phys. Rev. D 86, 114039 (2012).
[13] S. J. Brodsky, B. Kopeliovich, I. Schmidt and J. Soffer, Phys. Rev. D73, 113005 (2006).
[14] R. Pasechnik, B. Z. Kopeliovich and I. K.Potashnikova, arXiv:1403.2014 [hep-‐ph].
[15] B. Z. Kopeliovich, H. J. Pirner, I. K. Potashnikova, K. Reygers and I. Schmidt, Phys. Rev. D 88, no. 11, 116002 (2013).
[16] G. Aad et al. [ATLAS Collaboration], Phys. Rev. Lett. 110, 182302 (2013).
[17] Phys. Lett. B 725, 60 (2013)
[18] G. Aad et al. [ ATLAS Collaboration], arXiv:1409.1792 [hep-‐ex]; to ap-‐ pear in Phys. Rev. C.
[19] B. Z. Kopeliovich, H. J. Pirner, A. H. Rezaeian and I. Schmidt, Phys. Rev. D 77, 034011 (2008).
[20] B. Z. Kopeliovich, A. H. Rezaeian and I. Schmidt, Phys. Rev. D 78, 114009 (2008).
[21] B. Z. Kopeliovich, I. K. Potashnikova and I. Schmidt, Nucl. Phys. A 864, 203 (2011).
[22] T. Matsui and H. Satz, Phys. Lett. B 178, 416 (1986).
[23] B. Z. Kopeliovich, I. K. Potashnikova, I. Schmidt and M. Siddikov, Nucl. Phys. A (2014) [arXiv:1407.8080 [nucl-‐th]]; arXiv:1409.5147 [hep-‐ph].
[24] B. Z. Kopeliovich, I. K. Potashnikova and I. Schmidt, Phys. Rev. C 83, 021901 (2011).
[25] B. Z. Kopeliovich, J. Nemchik, I. K. Potashnikova and I. Schmidt, Phys. Rev. C 86, 054904 (2012).
[26] B. Z. Kopeliovich, I. K. Potashnikova and I. Schmidt, Phys. Rev. C 82, 037901 (2010).
[27] G. Aad et al. [ATLAS Collaboration], Phys. Lett. B 719, 220 (2013).
[28] G. Aad et al. [ATLAS Collaboration], arXiv:1408.5778 [hep-‐ex].
[29] B. Z. Kopeliovich, I. K. Potashnikova, B. Povh and E. Predazzi, Phys. Rev. Lett. 85, 507 (2000). 7
[30] B. Z. Kopeliovich, I. K. Potashnikova, B. Povh and E. Predazzi, Phys. Rev. D 63, 054001 (2001).
[31] B. Z. Kopeliovich, I. K. Potashnikova and B. Povh, Phys. Rev. D 86, 051502 (2012).
[32] B. Z. Kopeliovich, I. K. Potashnikova, B. Povh and I. Schmidt, Phys. Rev. D 76, 094020 (2007).
[33] B. Z. Kopeliovich and A. V. Tarasov, Phys. Lett. B 497, 44 (2001).
[34] E. A. Kuraev, L. N. Lipatov, and F. S. Fadin, Sov. Phys. JETP 45 (1977) 199; Ya. Ya. Balitsky and L. N. Lipatov, Sov. J. Nucl. Phys. 28 (1978) 22.
[35] L. V. Gribov, E. M. Levin and M. G. Ryskin, Phys. Rept.100 (1983) 1.
[36] L. D. McLerran and R. Venugopalan, Phys. Rev. D 49, 2233 (1994); Phys. Rev. D 49, 3352 (1994); Phys. Rev. D 50, 2225 (1994); E.Iancu, A. Leonidov and L. McLerran,Nucl. Phys. A 692, 583 (2001).
[37] I. Balitsky, Nucl. Phys. B463, 99 (1996); Phys. Rev. Lett. 81 2024 (1998); Phys. Rev.D60 014020 (1999).
[38] J. Jalilian Marian, A. Kovner, A.Leonidov and H. Weigert, Nucl. Phys.B504 415 (1997); Phys. Rev. D59 014014 (1999).
[39] Y. V. Kovchegov, Phys. Rev. D 61, 074018 (2000).
[40] E. Levin, L. Lipatov and M. Siddikov, Phys. Rev. D 89 (2014) 074002.
[41] E. Levin and S. Tapia, JHEP 1307 (2013) 183.
[42] T. Altinoluk, N. Armesto, A. Kovner, E. Levin and M. Lublinsky, JHEP 1408 (2014) 007.
[43] T. Altinoluk, A. Kovner, E. Levin and M. Lublinsky, JHEP 1404 (2014) 075.
[44] E. Levin, JHEP 1311 (2013) 039.
[45] T. Altinoluk, C. Contreras, A. Kovner, E. Levin, M. Lublinsky and A. Shulkin, JHEP 1309 (2013) 115.
[46] C. Contreras, E. Levin and R. Meneses, JHEP 1304 (2013) 085.
[47] G. Aad et al. [ATLAS Collaboration], arXiv:1408.4342 [hep-‐ex].
[48] E. Levin and A. H. Rezaeian, Phys. Rev. D 84, 034031 (2011).
[49] R. L. Ray, arXiv:1406.2736 [hep-‐ph].
[50] G. Aad et al. [ATLAS Collaboration], JHEP 1207, 019 (2012).
[51] G. Aad et al. [ATLAS Collaboration], Phys. Lett. B 725, 60 (2013).
[52] G. Aad et al. [ATLAS Collaboration], Phys. Rev. C 86, 014907 (2012).
[53] G. Aad et al. [ATLAS Collaboration], Phys. Rev. D 83, 052005 (2011)
[54] G. Aad et al. [ATLAS Collaboration], Phys. Rev. D 85, 012003 (2012); JHEP 1301, 086 (2013).
[55] J. Jalilian-‐Marian and A. H. Rezaeian, Phys. Rev. D86, 034016 (2012).
[56] A. H. Rezaeian, Phys. Rev. D86, 094016(2012).
[57] B. Z. Kopeliovich, A. H. Rezaeian, H. J. Pirner and I. Schmidt, Phys. Lett. B653, 210 (2007); Phys. Rev. D77, 034011 (2008); B. Z. Kope-‐ liovich, A. H. Rezaeian and I. Schmidt, Nucl. Phys. A807, 61 (2008); B. Z. Kopeliovich, E. Levin, A. H.Rezaeian and I. Schmidt, Phys. Lett. B675, 190 (2009); A. H. Rezaeian and A. Schaefer, Phys. Rev. D81, 114032 (2010).
[58] A. Kovner and A. H. Rezaeian, Phys. Rev. D90, 014031 (2014).
[59] A. H. Rezaeian and I. Schmidt, Phys. Rev. D88, 074016 (2013).
[60] N. Armesto and A. H. Rezaeian, Phys. Rev. D90, 054003 (2014).